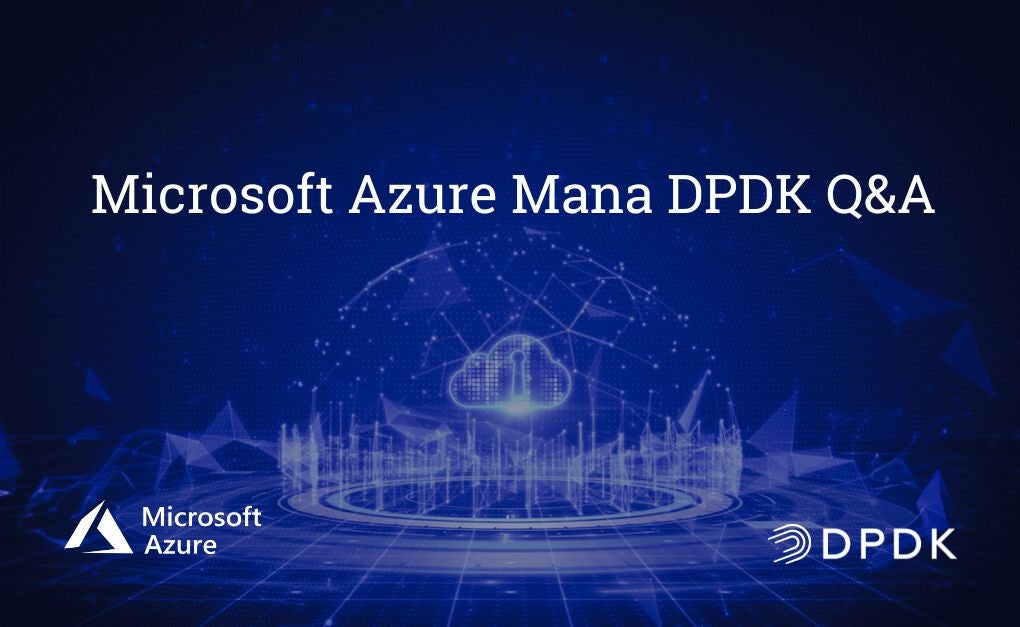
In today's rapidly evolving digital landscape, the demand for high-speed, reliable, and scalable network solutions is greater than ever. Enterprises are constantly seeking ways to optimize their network performance to…
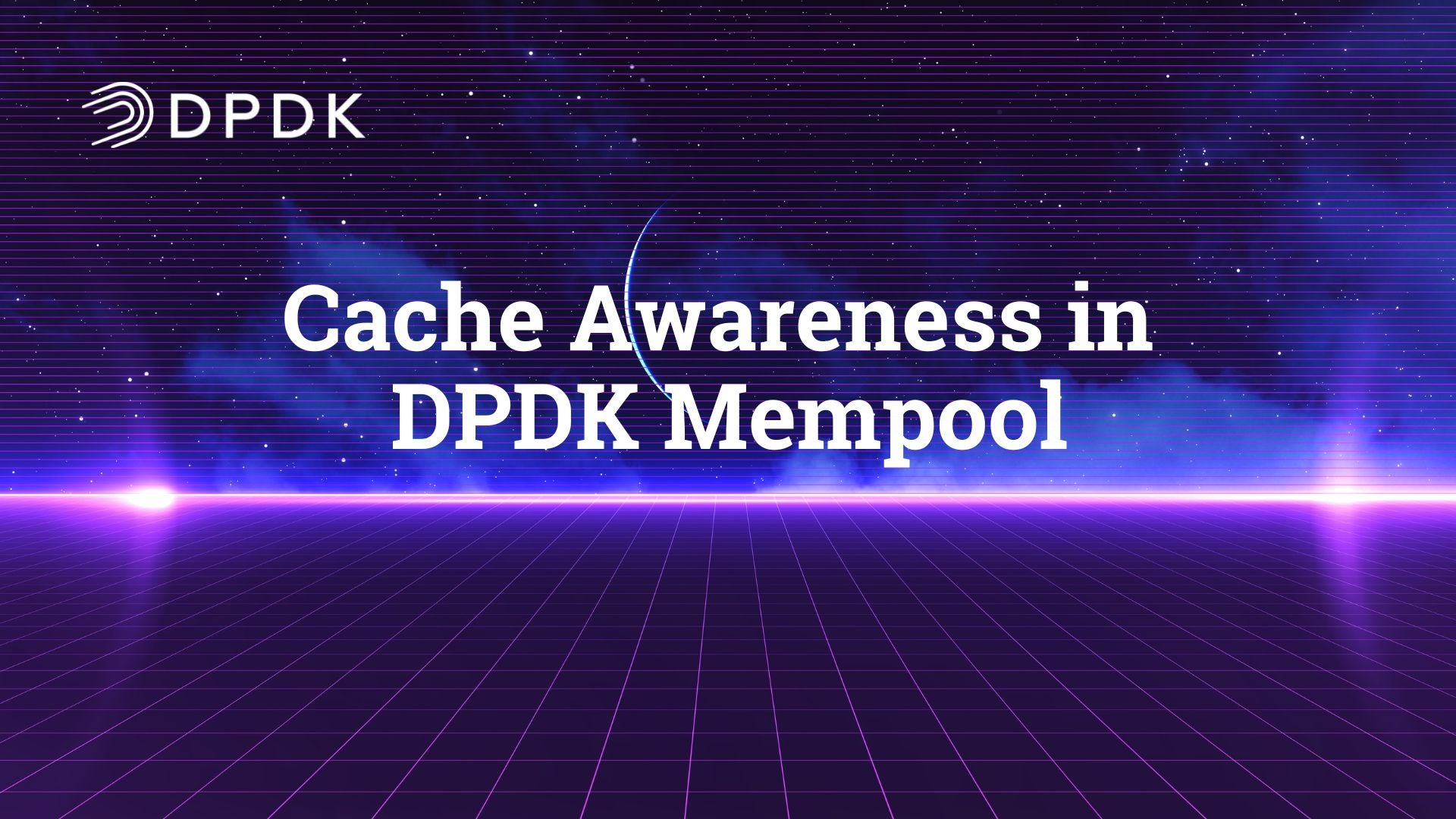
Author: Kamalakshitha Aligeri - Senior Software Engineer at Arm The objective of DPDK is to accelerate packet processing by transferring the packets from the NIC to the application directly, bypassing…
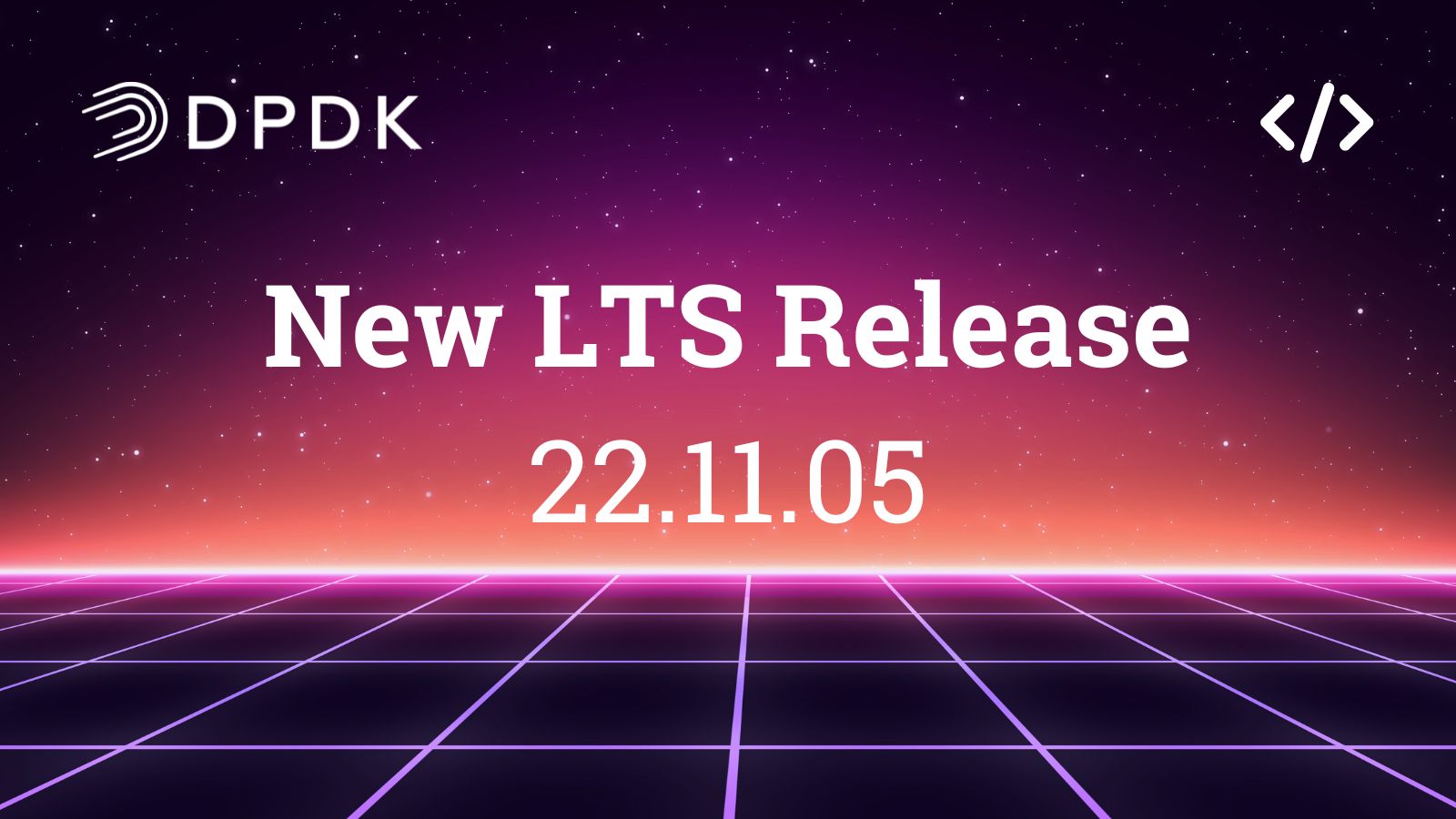
The latest DPDK Long Term Stable (LTS) Release 22.11.05 includes several updates and enhancements across various components of the DPDK framework. Significant changes in this release involve numerous file modifications,…
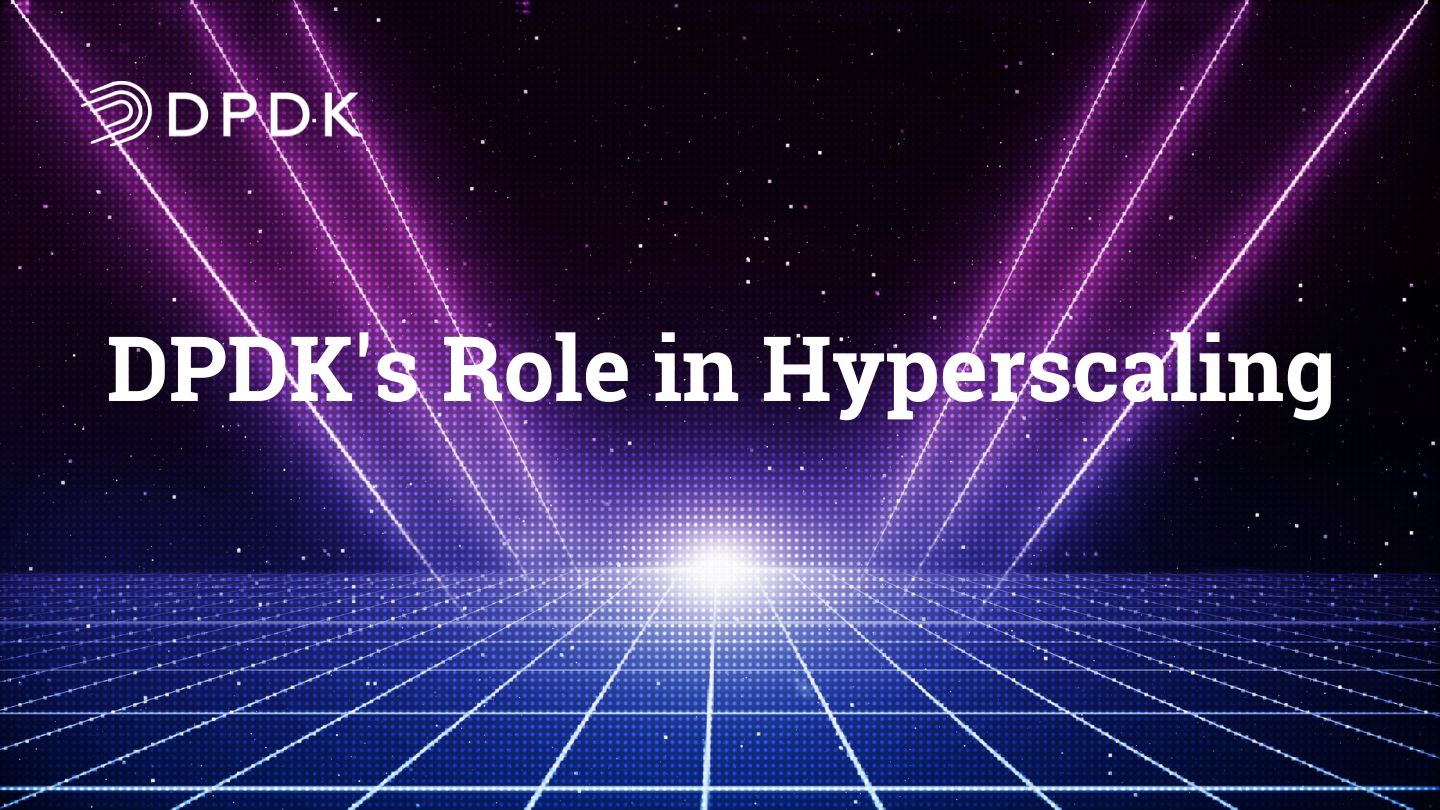
In the rapidly evolving digital landscape, hyperscaling in the cloud has emerged as a critical strategy for businesses aiming to scale their operations efficiently. The webinar, "Hyperscaling in the Cloud,"…

The latest DPDK release, version 22.11.4, brings several important updates, bug fixes, and improvements across various components of the DPDK framework. In this blog post, we'll provide a brief summary…

Navigating the Data Plane Development Kit (DPDK) release landscape requires a thorough understanding of what a Long-Term Stable (LTS) release entails and why it may be the preferred choice for…

Authors: Ben Thomas (Linux Foundation Marketing Lead) & Kevin Traynor (LTS Maintainer & Software Engineer @ Redhat) Navigating the complex landscape of Data Plane Development Kit (DPDK) releases, particularly Long-Term…

Introduction:The latest DPDK version 23.11, is now available. This version includes latest updates and new features in network processing. Download Link: https://fast.dpdk.org/rel/dpdk-23.11.tar.xz Release Statistics: Commits: This release comprises 1161 commits…
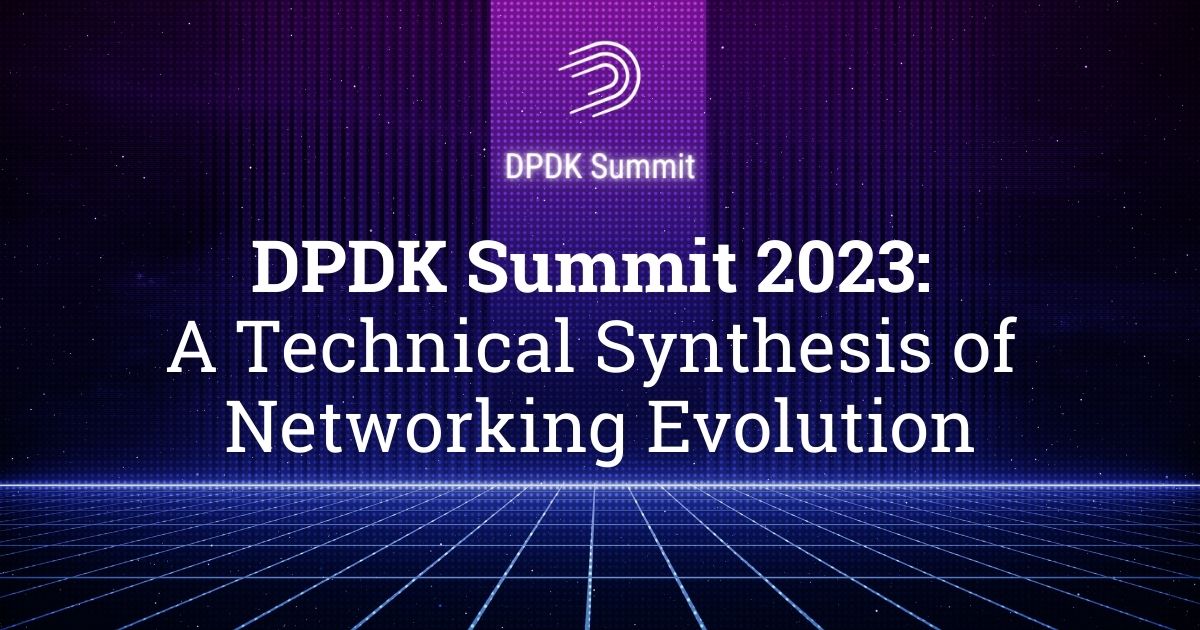
The DPDK Summit 2023 was a showcase of technical breakthroughs and forward-looking discussions in the field of high-performance networking. The summit featured a range of presentations, each diving into new…

Introduction The open-source community thrives on collective efforts to improve and augment software products. In this post, we take a closer look at the operations of the Data Plane Development…

We're excited to share with you the news that a new major release, DPDK 23.07, is now available for download. Here, we will take a detailed look at what this…
Hosted by The University of New Hampshire InterOperability Lab (UNH-IOL), the DPDK Community Lab exists to provide prompt and reliable continuous integration(CI) testing for all new patches submitted to DPDK…
A new major release, DPDK 22.11, is now available: https://fast.dpdk.org/rel/dpdk-22.11.tar.xz It was a comfortable release cycle, with: 1387 commits from 193 authors 1902 files changed, 137224 insertions(+), 61256 deletions(-) The…
The latest DPDK release, 22.07, is now available: https://fast.dpdk.org/rel/dpdk-22.07.tar.xz As is atypical, this Summer release is quite small: 1021 commits from 177 authors 1149 files changed, 77256 insertions(+), 26288 deletions(-)…
A new release is available: https://fast.dpdk.org/rel/dpdk-22.03.tar.xz Winter release numbers are quite small, as usual: 956 commits from 181 authors 2289 files changed, 83849 insertions(+), 97801 deletions(-) There are no plans…
By David Marchand A new major release of DPDK, DPDK 21.11, is now available: https://fast.dpdk.org/rel/dpdk-21.11.tar.xz This is a big DPDK release, with: 1875 commits from 204 authors 2413 files changed,…
By Thomas Monjalon, DPDK Tech Board The DPDK community has issued its latest quarterly release, 21.08, which is available here: https://fast.dpdk.org/rel/dpdk-21.08.tar.xz Stats for this (smaller) release: 922 commits from 159…
The DPDK (https://www.dpdk.org) project presents a unique set of challenges for fully testing the code, with the largest coverage possible (i.e. the goal of continuous integration and gating). The DPDK…
By Honnappa Nagarahalli As an increasingly higher number of cores are packed together in a SoC, thread synchronization plays a key role in scalability of the application. In the context…
By Thomas Monjalon, DPDK Tech Board A new DPDK release is now available, 21.05: https://fast.dpdk.org/rel/dpdk-21.05.tar.xz It was a quite big cycle, as is typical with our May releases: 1352 commits…
We are thrilled to announce that our recent DPDK Summit APAC was the first Linux Foundation event to have earned a Gold Diversity & Inclusion badge from the Linux Foundation’s…
By Honnappa Nagarahalli DPDK is widely used across the technology industry to accelerate packet processing on a varied mix of platforms and architectures. However, all architectures are not created the…
By Thomas Monjalon from the DPDK Tech Board The DPDK community has issued a new release, 21.02: https://fast.dpdk.org/rel/dpdk-21.02.tar.xz It was a light period as February releases of the 2 previous…
In DPDK 20.11, the DPDK community adopted ABI stable releases with the aim of easing the adoption of new features for DPDK users. If ABI stable releases is a new…
To learn more about Microsoft, DPDK’s newest Gold member, and how they are working within the DPDK community, we sat down with Omar Cardona, Doug Stamper and Harini Ramakrishnan from…
The DPDK Project has had an active third quarter thus far with the DPDK Userspace Summit in September, release of 20.11 release (the biggest DPDK release yet!), and the Governing…
A new major DPDK release is now available: https://fast.dpdk.org/rel/dpdk-20.11.tar.xz Our Thanksgiving gift to the ecosystem is the biggest DPDK release ever, with: 2195 commits from 214 authors 2665 files changed,…
For the past three years, the DPDK developer community has honored some of the most outstanding contributions across the project over the past year. While things are quite different this…
By Lincoln Lavoie, UNH-IOL, Principal Engineer A little more than 3 years ago, we set out to improve the qualities and capabilities of the continuous integration (CI) testing of the…
A new release is available: https://fast.dpdk.org/rel/dpdk-20.08.tar.xz This version could be named Twins: 20.08 is released on 2020-08-08.The other numbers are: 1175 commits from 159 authors 1199 files changed,…
The DPDK Project Governing board held a "virtual" meeting on July 13th, welcoming both Doug Stamper from Microsoft and Marvell's new representative, Prasun Kapoor, to the board. Bruce Richardson (of…
We hope you are keeping safe and healthy, as we all adjust to the new normal we find ourselves in, with coronavirus and social unrest around us. We’re proud of…
A new DPDK release, 20.05, is available here: https://fast.dpdk.org/rel/dpdk-20.05.tar.xz It was quite a big release cycle, with: 1304 commits from 189 authors 1983 files changed, 145825 insertions(+), 29147 deletions(-) There…
Greetings DPDK Project Community: We hope you are managing okay and remaining safe and healthy as the coronavirus crisis has thrown the world into a frenzy. While many of us…
The latest DPDK release, 20.02, is now available: https://fast.dpdk.org/rel/dpdk-20.02.tar.xz The statistics show a post-LTS ABI-compatible release: 820 commits from 156 authors 876 files changed, 59941 insertions(+),…
Our 2020 event planning is taking longer than usual, given the current situation with the Novel CoronaVirus. We are working diligently to solidify plans for events throughout 2020 and will…
A new major release is available: https://fast.dpdk.org/rel/dpdk-19.11.tar.xz The statistics are quite impressive: 1611 commits from 199 authors 1914 files changed, 164270 insertions(+), 44609 deletions(-) The branch 19.11 should be supported…
A Q&A with Rashid Khan, DPDK Board Member and Director, Networking at Red Hat Rashid Khan is the Director of Networking development at Red Hat. His team’s responsibilities include…
Ray Kinsella & Thomas Monjalon Most open-source software projects follow distinct life cycle patterns as they evolve from the genesis of a idea, all the way through to a mature…
The DPDK developer community convenes each year at the DPDK “Userspace” event to share knowledge, discuss best practices, and further align the community. During the event we take some…
By Antanoly Burakov This post is Part 2 of a 4-part blog series that was originally published on the Intel Developer Zone blog. Introduction In the previous article, we covered the…
By Antanoly Burakov This post is Part 1 of a 4-part blog series that was originally published on the Intel Developer Zone blog. Introduction Memory management is a core aspect…
The latest major release of DPDK is now available, DPDK 19.08: https://fast.dpdk.org/rel/dpdk-19.08.tar.xz. Arguably the biggest release of the year, DPDK 19.08 was a phenomenal community effort. The statistics - probably…
This post originally appeared on the Microsoft Tech Community (Networking) blog, here: https://techcommunity.microsoft.com/t5/Networking-Blog/DPDK-releases-v19-05-introduces-Windows-Support/ba-p/633927 By Harini Ramakrishnan Data Plane Development Kit recently issued the release of DPDK v19.05. We are thrilled to share that…
By Jeremy Plsek, Lincoln LaVoie and Patrick MacArthur The DPDK Community Lab is an open, independent testing resource for the DPDK project. Its purpose is to perform automated testing on…
As the DPDK community continues to make strides, we’d like to take some time to reflect upon successes of the past year and announce the winners of the inaugural DPDK…
Originally posted by Yuanhan Liu yuanhan.liu at linux.intel.com on the dpdk-stable mailing list Hi all, Please join me in announcing the first DPDK stable release: http://fast.dpdk.org/rel/dpdk-16.07.1.tar.xz It includes most bug fixing patches…
Originally posted by zimeiw zimeiw at 163.com on the dpdk-dev mailing list hi, The tcp/ip stack is developed based on dpdk. tcp/ip stack and APP deployment. |-------| |-------| |-------| | APP |…
Originally posted by Antti Kantee (pooka at iki.fi) on the dpdk-dev mailing list ---- Hi, I like the opportunities that a technology like DPDK enables, and I felt that the availability of…